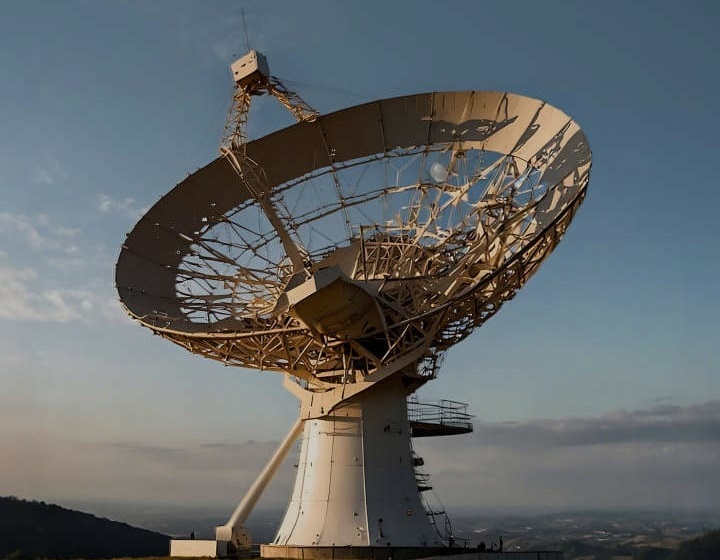
In a world where technology is getting smaller and smarter — think of smartphones, microchips, and ultra-slim laptops — it’s natural to wonder:
Why are satellite dishes still so huge?
You’ve probably seen massive satellite dishes towering over communication centers or mounted on high buildings, and even larger ones at space observatories. Despite all the progress in technology, the iconic large round dish remains an essential part of global communication and scientific exploration.
The truth is, the size of satellite dishes isn’t about outdated technology or inefficiency.
It’s the result of deep physical principles, technical challenges, and our insatiable desire to communicate across vast cosmic distances.
In this article, we'll explore why satellite dishes are so huge, touching on the science behind their design, the challenges they solve, and why, even in a high-tech world, bigger still means better when it comes to satellite communication.
At their most basic, satellite dishes are a type of antenna.
They are designed to either:
Receive signals from a satellite orbiting Earth
Send signals back to a satellite (called uplinking)
Instead of receiving nearby signals like a traditional radio antenna, satellite dishes deal with signals that have traveled tens of thousands of kilometers through space.
By the time a satellite’s signal reaches Earth, it’s:
Extremely weak (often less than a billionth of a watt)
Easily disrupted by weather, atmosphere, and noise
Thus, satellite dishes need to capture as much of that tiny signal as possible and focus it sharply onto a small receiver to make it usable.
The number one reason why satellite dishes are large is simple physics.
When a satellite transmits a signal, it spreads out in space like an ever-expanding balloon.
By the time it reaches Earth, the signal is incredibly spread out and weak.
Because of the inverse square law:
Signal strength decreases with the square of the distance.
Double the distance, and the signal becomes four times weaker.
Satellites orbit Earth at different heights:
Low Earth Orbit (LEO): About 500–2,000 km above Earth
Geostationary Orbit (GEO): About 36,000 km above Earth!
Communicating reliably with satellites that far away requires huge dishes to capture enough of the weakened signal.
The larger the dish:
The greater the surface area to collect the faint signals.
The higher the “gain” (signal amplification).
Fact:
A dish's ability to collect and focus signal energy is proportional to the square of its diameter.
This means:
Doubling the diameter quadruples the collecting power.
Tripling the diameter multiplies the collecting power by nine!
Thus, bigger dishes can capture more of the signal and focus it better, ensuring clearer communication.
Satellite dishes are parabolic reflectors.
Their bowl-like shape reflects incoming signals toward a small point — the feed horn — where the receiver is located.
The bigger the dish:
The narrower and sharper its focus.
The less interference it collects from surrounding noise.
This "sharpness" is known as beamwidth.
A narrow beamwidth means:
Better isolation of the desired satellite signal
Less noise from neighboring satellites or cosmic background
Think of it like adjusting the focus on a camera:
A big, well-focused lens captures a crisp image of a distant subject, while a small or poorly focused lens yields a blurry mess.
In crowded orbital zones where satellites are packed closely together, precision targeting is vital — another reason for large dishes.
Even after traveling through the vacuum of space, satellite signals must pass through Earth’s atmosphere, which can distort, weaken, or scatter them.
Challenges include:
Rain fade (signal loss due to rain)
Snow and ice interference
Clouds and humidity absorbing high-frequency signals
Higher frequency signals (like Ka-band used for satellite internet) are even more vulnerable to atmospheric effects.
How size helps:
A larger dish collects more of the weakened signal.
It maintains a stronger signal-to-noise ratio (SNR).
It ensures reliable communication even in poor weather conditions.
Thus, the bigger the dish, the better it can resist nature’s attempt to block the signal.
When dishes send signals back to satellites (uplinking), they must overcome:
Enormous distances
Atmospheric distortion
Interference limits
To focus enough power into a tight, directed beam:
A larger dish is needed to prevent the signal from "spreading out" too much.
It minimizes energy loss and interference with other satellites.
Ground stations — like those used for TV broadcasts, internet backbones, or scientific communication — often have dishes 10 to 30 meters across for this reason.
Without large dishes, signals would:
Scatter
Weaken
Fail to reach their targets
Satellite communication uses different parts of the electromagnetic spectrum:
C-band (4–8 GHz): Low frequency, needs bigger dishes but is less affected by rain.
Ku-band (12–18 GHz): Higher frequency, allows smaller dishes (used for many satellite TVs).
Ka-band (26–40 GHz): Even higher frequencies, enabling smaller dishes, but very sensitive to rain fade.
In short:
Lower frequencies = larger dishes.
Higher frequencies = potentially smaller dishes, but with trade-offs.
Thus, not all satellite dishes need to be huge — but the bigger dishes remain necessary for high reliability and critical communications, especially at lower frequencies.
When satellite communications first began:
Satellites were low-powered.
Receivers were primitive by today's standards.
Thus, massive dishes were essential.
Examples:
Early Bird (Intelsat I, 1965):
Needed Earth stations with dishes up to 30 meters wide.
Telstar (1962):
Required a 53-meter dish to receive its weak signals from space.
Over time, technology improved:
Satellites transmitted stronger signals.
Receivers became more sensitive.
Compression technologies (like digital encoding) reduced bandwidth needs.
Thus, household satellite dishes shrank — but large dishes are still critical for:
Space missions
Transcontinental internet backbones
Military and scientific communications
For space exploration missions — think Mars rovers, Voyager spacecraft, James Webb Space Telescope — dishes must be absolutely massive.
Examples:
NASA’s Deep Space Network (DSN):
Uses 70-meter (230-foot) dishes to maintain contact with spacecraft billions of kilometers away.
Arecibo Observatory (Puerto Rico):
(before its collapse) — had a 305-meter (1,000-foot) dish!
In these cases, there’s simply no replacement for size.
The signals are so faint that only huge collecting areas can detect them.
Emerging technologies are trying to reduce the need for gigantic dishes:
Phased array antennas:
Flat panels that electronically steer the beam without moving parts (e.g., Starlink).
Smart tracking systems:
Keep smaller dishes precisely aligned with fast-moving satellites.
However:
For deep space communication
For extremely high data rate transmission
For ultra-secure government and military uses
Large parabolic dishes remain irreplaceable for now.
Physics — particularly the need to collect and focus extremely weak signals — hasn't changed.
In the world of satellite communication, size isn't about inefficiency.
It’s a reflection of:
The vastness of space
The weakness of distant signals
The unchanging laws of physics
Big dishes mean:
Better signal collection
Sharper focus
Stronger transmission power
Resilience against weather and interference
Far from being relics of a bygone era, huge satellite dishes are still crucial bridges between Earth and the universe — tools that make global communication, space exploration, and scientific discovery possible.
And sometimes, to touch the stars, you need a really big hand.